Conductive hydrogels are innovative materials that have gained significant attention in the field of biomedical engineering. In this article, we will dive into the basics of conductive hydrogels, explore their composition and importance, examine the science behind them, learn about the manufacturing process, discuss their various applications, and evaluate both their advantages and limitations. So, let's start by understanding the fundamentals of conductive hydrogels.
โ
Understanding the Basics of Conductive Hydrogels
โ
Definition and Composition
โ
Conductive hydrogels are three-dimensional networks of hydrophilic polymers that are capable of conducting electricity. They consist of polymer chains, typically made of polymers such as polyacrylamide or polyvinyl alcohol, which are cross-linked together to form a gel-like structure. These hydrogels have the ability to retain large amounts of water due to their high water content, which makes them similar to natural tissues in terms of their physical properties.
โ
When it comes to the composition of conductive hydrogels, it is important to note that the choice of polymers and cross-linking agents can greatly influence their electrical conductivity. For example, the addition of conductive nanoparticles, such as carbon nanotubes or graphene, can enhance the electrical properties of the hydrogel. These nanoparticles provide a conductive pathway within the gel structure, allowing for the efficient flow of electrons.
โ
Importance in Biomedical Engineering
โ
Conductive hydrogels have become vital in the field of biomedical engineering due to their unique characteristics and versatility. These hydrogels exhibit electrical conductivity, which enables them to serve as effective materials for developing various biomedical devices and platforms.
โ
One of the key applications of conductive hydrogels is in the field of bioelectrodes. These hydrogels can be used to create flexible and biocompatible electrodes that can interface with biological tissues. This is particularly useful in applications such as electrocardiography (ECG) and electroencephalography (EEG), where the electrodes need to be in direct contact with the skin to measure electrical signals.
โ
Biosensors are another area where conductive hydrogels have found significant use. By incorporating specific biological receptors or enzymes into the hydrogel matrix, these sensors can detect and quantify various analytes, such as glucose or pH levels, in biological samples. The electrical conductivity of the hydrogel allows for the transduction of the detected signals, providing real-time measurements.
โ
In addition to bioelectrodes and biosensors, conductive hydrogels are also being explored for drug delivery systems. The ability of these hydrogels to retain large amounts of water and their biocompatibility make them ideal candidates for controlled release of drugs. By incorporating drug molecules into the hydrogel matrix, the release can be regulated by external stimuli, such as electrical or pH changes, offering a targeted and controlled drug delivery approach.
โ
Furthermore, conductive hydrogels have shown great promise in tissue engineering. Their ability to support cell growth and mimic the physical properties of natural tissues make them valuable scaffolds for tissue regeneration. By incorporating conductive nanoparticles into the hydrogel, it is possible to create an environment that promotes cell adhesion, proliferation, and differentiation. This opens up possibilities for the development of functional tissues, such as cardiac or neural tissues, that can integrate with the host body.
โ
โ

โ
โ
โ
The Science Behind Conductive Hydrogels
โ
Conductive hydrogels have gained significant attention in the field of materials science due to their unique properties and potential applications. These hydrogels exhibit both chemical and physical properties that make them highly desirable for a wide range of uses.
โ
Chemical Properties
โ
The conductive properties of hydrogels can be attributed to the incorporation of conductive additives or the introduction of functional groups that enhance their electrical conductivity. Common conductive additives include carbon nanotubes, graphene, and metallic nanoparticles. These additives create a conductive pathway within the hydrogel network, allowing the flow of electrical charges.
โ
Carbon nanotubes, for example, are one of the most widely used conductive additives in hydrogel research. These cylindrical structures composed of carbon atoms possess exceptional electrical conductivity due to their unique arrangement of carbon atoms. When incorporated into hydrogels, carbon nanotubes form a conductive network that enables the efficient transport of electrons.
โ
Graphene, on the other hand, is a two-dimensional material consisting of a single layer of carbon atoms arranged in a hexagonal lattice. Its high electrical conductivity and mechanical strength make it an ideal additive for enhancing the conductive properties of hydrogels. When graphene is dispersed within a hydrogel matrix, it forms a conductive network that facilitates the movement of electrical charges.
โ
Metallic nanoparticles, such as silver or gold nanoparticles, are also commonly used as conductive additives in hydrogels. These nanoparticles possess excellent electrical conductivity and can be easily incorporated into hydrogel networks. The presence of metallic nanoparticles within the hydrogel structure allows for the efficient transfer of electrical charges, making the hydrogel conductive.
โ
โ

โ
โ
Physical Properties
โ
In addition to their chemical properties, conductive hydrogels possess a unique combination of mechanical and electrical properties. They are soft and flexible, similar to human tissues, which makes them suitable for applications requiring conformability and flexibility. This characteristic is particularly advantageous in biomedical applications, where hydrogels can be used as scaffolds for tissue engineering or as wearable sensors.
โ
Furthermore, conductive hydrogels have a high water content, which promotes good biocompatibility. The high water content allows for efficient diffusion of nutrients and oxygen, making them suitable for applications in tissue engineering and drug delivery. The hydrogel's ability to retain water also contributes to its soft and flexible nature, providing a comfortable environment for cells and tissues.
โ
Moreover, the electrical conductivity of conductive hydrogels opens up new possibilities for applications in bioelectronics. By integrating electronic components into the hydrogel matrix, it is possible to create bioelectronic devices that can interface with biological systems. These devices can be used for various purposes, such as monitoring physiological signals, delivering electrical stimulation, or even controlling the release of drugs.
โ
Manufacturing Process of Conductive Hydrogels
โ
Conductive hydrogels have gained significant attention in recent years due to their unique properties and potential applications in various fields, including biomedical engineering, flexible electronics, and energy storage. The manufacturing process of conductive hydrogels involves a series of carefully orchestrated steps to ensure the desired properties and performance of the final product.
โ
Materials Required
โ
The manufacturing process of conductive hydrogels typically involves the use of water-soluble polymers, conductive additives, and cross-linking agents. Water-soluble polymers, such as polyvinyl alcohol (PVA) or polyethylene glycol (PEG), serve as the backbone of the hydrogel matrix. These polymers provide the necessary structural integrity and water absorption capacity. Conductive additives, such as carbon nanotubes or graphene, are incorporated into the hydrogel matrix to impart electrical conductivity. Cross-linking agents, such as glutaraldehyde or genipin, are used to form a three-dimensional network by chemically linking the polymer chains together. Depending on the desired properties and applications, various combinations of these materials can be used.
โ
Step-by-Step Guide
โ
The manufacturing process of conductive hydrogels generally involves the following steps:
- 1. Dissolution of the water-soluble polymer in a solvent.
The first step in the manufacturing process is the dissolution of the water-soluble polymer in a suitable solvent. This step ensures that the polymer chains are evenly dispersed and ready for further processing. The choice of solvent depends on the specific polymer used and its solubility characteristics. Common solvents include water, ethanol, or a mixture of both.
- 2. Addition of conductive additives and mixing until homogeneity is achieved.
Once the polymer is dissolved, conductive additives, such as carbon nanotubes or graphene, are added to the solution. These additives play a crucial role in enhancing the electrical conductivity of the hydrogel. The mixture is then thoroughly mixed or sonicated to ensure the uniform dispersion of the conductive additives throughout the polymer solution. This step is crucial to achieve a homogenous distribution of conductive particles, which is essential for the overall performance of the hydrogel.
- 3. Cross-linking of the polymer chains to form the hydrogel network using a cross-linking agent.
After achieving a homogenous mixture, a cross-linking agent is introduced to initiate the cross-linking reaction. The cross-linking agent reacts with the functional groups present on the polymer chains, forming covalent bonds and creating a three-dimensional network structure. This cross-linking process is typically carried out under controlled conditions, such as specific temperature and pH, to ensure the desired cross-linking density and mechanical properties of the hydrogel.
- 4. Removal of the solvent to obtain the final conductive hydrogel.
Once the cross-linking reaction is complete, the solvent used in the initial polymer dissolution step needs to be removed. This can be achieved through various methods, such as evaporation, freeze-drying, or solvent exchange. The removal of the solvent is crucial to obtain the final conductive hydrogel with the desired properties. Care must be taken to ensure that the hydrogel structure remains intact during the solvent removal process.
โ
The manufacturing process of conductive hydrogels is a delicate balance of material selection, formulation, and processing parameters. Each step plays a crucial role in determining the final properties of the hydrogel, such as electrical conductivity, mechanical strength, and water absorption capacity. By carefully controlling these steps, researchers and engineers can tailor the properties of conductive hydrogels to meet specific application requirements, opening up a wide range of possibilities for their use in various industries.
โ
Applications of Conductive Hydrogels
โ
Use in Medical Devices
โ
Conductive hydrogels play a crucial role in the development of medical devices such as bioelectrodes and biosensors. These hydrogels act as interfaces between the device and biological tissues, allowing for seamless communication and accurate monitoring of physiological signals. They enable the detection of various biomarkers and provide a stable and biocompatible platform for long-term use.
โ
โ
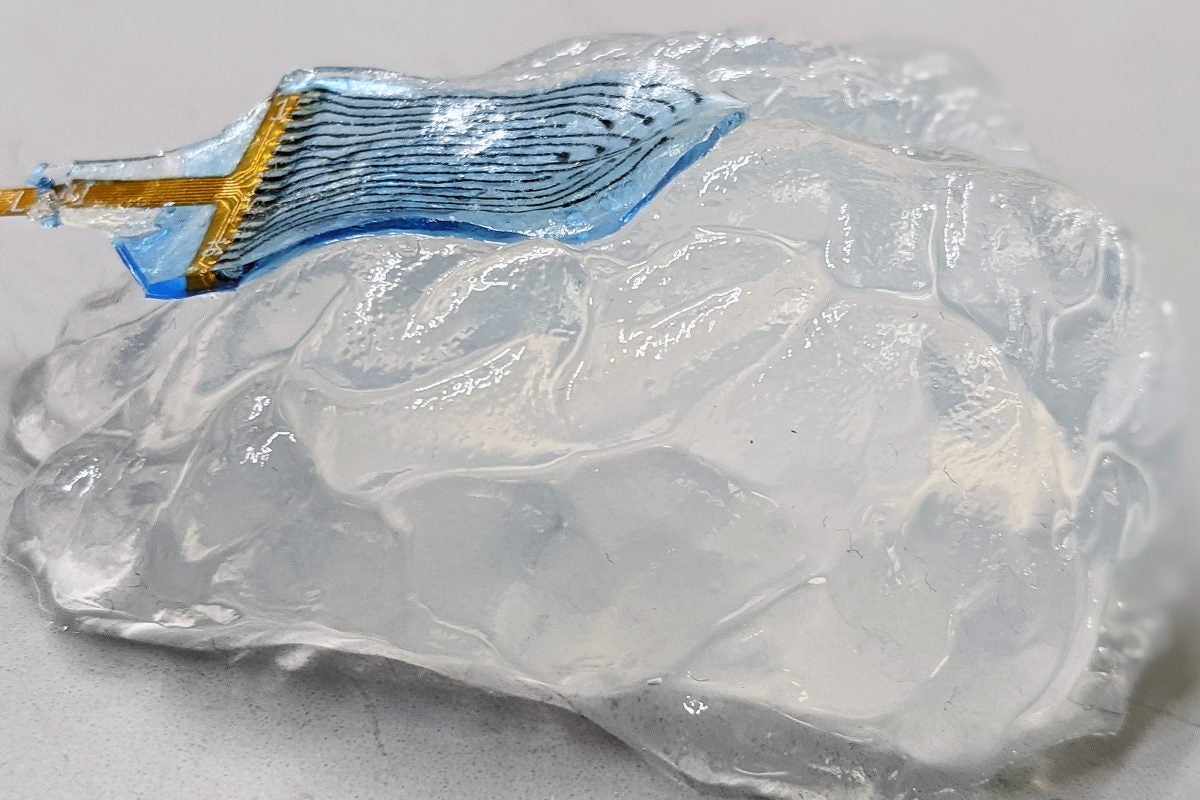
โ
โ
Role in Tissue Engineering
โ
Conductive hydrogels have revolutionized the field of tissue engineering by providing a scaffold-like structure that supports cell growth and tissue regeneration. These hydrogels can be functionalized with specific cues to guide cell behavior and promote tissue differentiation. Furthermore, their electrical conductivity can be harnessed to stimulate tissue growth and enhance functional restoration.
โ
Advantages and Limitations of Conductive Hydrogels
โ
Benefits of Using Conductive Hydrogels
โ
Conductive hydrogels offer several advantages over traditional materials in biomedical applications. They provide a biocompatible and flexible platform that closely mimics the mechanical and electrical properties of natural tissues. The ability to tailor their properties, such as conductivity and swelling behavior, allows for customization based on specific application requirements. Moreover, their high water content promotes cell viability and nutrient exchange.
โ
Challenges and Potential Solutions
โ
Despite their promising characteristics, conductive hydrogels do face some challenges. The incorporation of conductive additives can affect the mechanical strength and biodegradability of the hydrogels, which may limit certain applications. Additionally, achieving uniform conductivity throughout the hydrogel can be challenging. However, ongoing research aims to address these limitations through the development of novel manufacturing techniques and the design of innovative composite materials.
โ
Conclusion
โ
Conductive hydrogels have emerged as valuable materials in the field of biomedical engineering. Their unique properties, such as electrical conductivity and biocompatibility, make them ideal for various applications ranging from medical devices to tissue engineering. While there are still challenges to overcome, ongoing advancements in manufacturing processes and material design hold great promise for the future of conductive hydrogels in healthcare and beyond.