Fusion reactors have intrigued scientists and engineers for decades as a potential source of clean and abundant energy. But how do they work? In this article, we'll explore the principles behind nuclear fusion and the different types of fusion reactors.
Understanding Nuclear Fusion
Nuclear fusion is the process of combining two atomic nuclei to form a heavier nucleus. This process releases a tremendous amount of energy in the form of light and heat. Fusion reactions occur naturally in the sun and other stars, where hydrogen atoms combine to create helium.
However, scientists are working to replicate this process on Earth to create a new source of renewable energy. The challenge lies in controlling the fusion reaction and harnessing its power.
While nuclear fission has been the primary source of nuclear energy for decades, it has several drawbacks, including the risk of nuclear accidents and the production of radioactive waste. Fusion, on the other hand, has the potential to be a much safer and cleaner source of energy.
What is Nuclear Fusion?
Nuclear fusion occurs when the energy released by a fusion reaction is greater than the energy required to initiate the reaction. This energy is produced by the collisions between atomic nuclei. To achieve fusion on Earth, scientists need to create the right conditions for these collisions to occur.
They do this by creating a high-temperature plasma of hydrogen isotopes. The plasma is heated to millions of degrees using lasers, magnetic fields, or other methods until the hydrogen nuclei become so energetic that they overcome their electrical repulsion and collide, releasing energy.
The process of nuclear fusion is incredibly complex and requires a deep understanding of plasma physics, material science, and nuclear engineering. Scientists have been working on fusion research for decades and have made significant progress, but there is still much work to be done before fusion can become a viable source of energy.
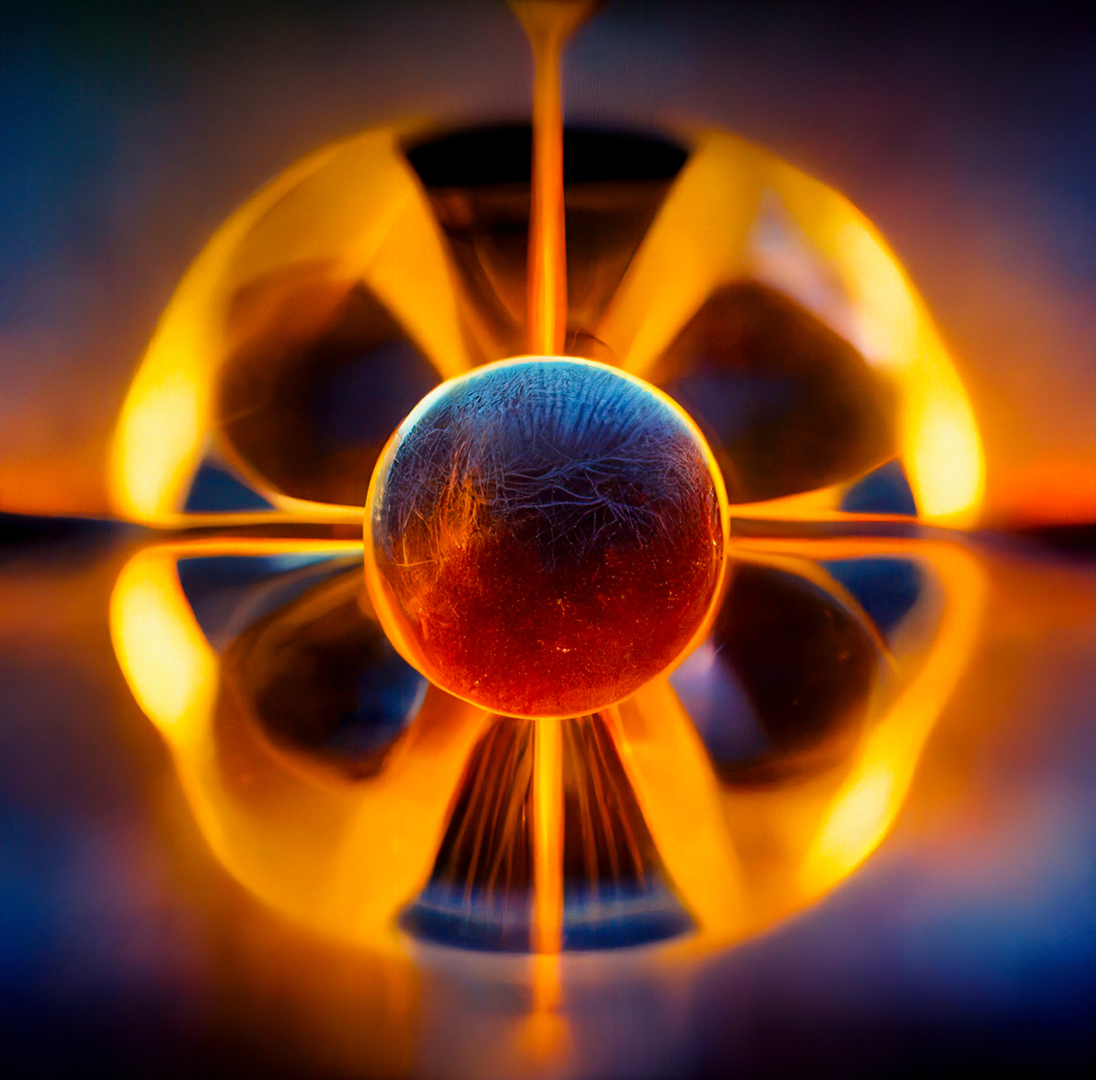
Fusion vs. Fission: The Key Differences
Fusion is often compared to nuclear fission, the process used in traditional nuclear reactors. While fission involves splitting a heavy nucleus into smaller ones, fusion involves merging lighter nuclei into a heavier one.
The advantages of fusion are numerous. It produces no greenhouse gases or other pollutants, and it requires much less fuel than fission. Additionally, fusion reactions cannot result in a runaway reaction or meltdown, making them much safer than fission.
Another significant advantage of fusion is the potential to use widely available resources as fuel. Deuterium, an isotope of hydrogen, can be extracted from seawater, and tritium can be produced from lithium, which is abundant in the Earth's crust. This means that fusion could provide a nearly limitless source of energy.
The Science Behind Fusion Reactions
The science of fusion reactions is complex and multifaceted. It involves a deep understanding of plasma physics, material science, and nuclear engineering. The goal is to create a sustainable and stable fusion reaction that produces more energy than it consumes.
At the core of a fusion reactor is the plasma chamber, a vessel designed to contain a high-temperature plasma of hydrogen isotopes. This plasma is subject to intense magnetic fields that keep it in place and prevent it from contacting the chamber's walls.
One of the biggest challenges facing fusion research is the need to create a self-sustaining reaction. In a self-sustaining reaction, the energy produced by the fusion reaction is enough to maintain the high temperature and pressure needed for the reaction to continue. Achieving this is known as achieving ignition, and it is the ultimate goal of fusion research.
While there are still many challenges to overcome, scientists are optimistic about the potential of fusion energy. With continued research and development, fusion could provide a safe, clean, and nearly limitless source of energy for generations to come.

The Components of a Fusion Reactor
Let's take a closer look at the different components that make up a fusion reactor. A fusion reactor is a complex machine that requires careful design and construction to achieve the high temperatures and pressures necessary for fusion to occur.
The Plasma Chamber
The plasma chamber is the heart of a fusion reactor. It is where the fusion reaction takes place, and it needs to be carefully designed and constructed to withstand the intense heat and pressure generated by the plasma. The plasma is a highly energetic gas made up of charged particles that are heated to millions of degrees.
The walls of the chamber are typically made of high-quality materials like tungsten or carbon fiber to resist erosion and damage caused by plasma interactions. The chamber itself is designed to trap the plasma and keep it at high pressure to facilitate fusion reactions.
Magnetic Confinement Systems
Magnetic confinement systems are used to keep the plasma in place and prevent it from coming into contact with the walls of the chamber. These systems use powerful magnetic fields to trap and control the plasma, allowing it to reach the high temperatures and densities necessary for fusion to occur.
The magnetic fields are created by superconducting coils that are cooled to extremely low temperatures using liquid helium. These coils generate a magnetic field that is strong enough to contain the plasma, but also flexible enough to allow for adjustments to the plasma shape and position.
Heating Systems
The plasma in a fusion reactor needs to be heated to millions of degrees to initiate the fusion reaction. Different heating systems can be used, such as lasers, radio waves, or particle beams. These heating systems are carefully designed to deliver energy to the plasma in a controlled and efficient manner.
One of the most promising heating techniques is called neutral beam injection. This involves using a beam of neutral atoms to transfer energy to the plasma. The neutral atoms are created by stripping electrons from hydrogen gas and then accelerating the resulting ions to high speeds. When these ions collide with a surface, they release neutral atoms that can then be directed into the plasma.
Cooling Systems
As with any high-temperature system, cooling is critical to the safe and efficient operation of a fusion reactor. Different cooling systems can be used, such as water-cooled panels or liquid metal coolants. The cooling systems are designed to remove heat from the reactor and transfer it to a heat exchanger, where it can be used to generate electricity.
One of the most promising cooling techniques is called helium cooling. This involves using liquid helium to cool the superconducting coils that generate the magnetic fields. The helium is circulated through the coils and then cooled using a cryocooler. This allows the coils to operate at extremely low temperatures without losing their superconductivity.
Diagnostic and Control Systems
Fusion reactors require sophisticated diagnostic and control systems to monitor and adjust their operations. These systems use sensors and computer algorithms to detect changes in the plasma and make adjustments to maintain stable and sustainable fusion reactions.
One of the key diagnostic tools used in fusion reactors is called a Langmuir probe. This is a small electrode that is inserted into the plasma and used to measure the plasma density and temperature. This information is then used to adjust the heating and cooling systems to maintain the desired plasma conditions.
In addition to diagnostic tools, fusion reactors also require advanced control systems to regulate the flow of fuel into the reactor and adjust the magnetic fields to maintain stable plasma conditions. These control systems are typically based on complex computer algorithms that are designed to optimize reactor performance while maintaining safety and reliability.
.jpeg)
Types of Fusion Reactors
There are many different types of fusion reactors, each with its unique strengths and challenges. Let's take a closer look at some of the most common types.
Tokamak Reactors
Tokamak reactors are the most widely studied type of fusion reactor. They use doughnut-shaped chambers to trap and control a toroidal plasma.
Tokamaks are attractive because they are relatively easy to build and operate, but they face challenges in achieving the necessary plasma temperatures and densities.
One of the biggest challenges in creating a successful tokamak reactor is finding a way to sustain the plasma for long periods of time. The plasma must be heated to temperatures hotter than the core of the sun and must be sustained for long enough to allow the fusion reactions to occur.
Despite these challenges, tokamak reactors have made significant progress in recent years. The International Thermonuclear Experimental Reactor (ITER) is currently under construction in France and is expected to be the first tokamak reactor to achieve sustained fusion reactions.

Stellarator Reactors
Stellarator reactors use complex systems of magnetic fields to control the plasma, avoiding some of the issues faced by tokamaks.
Their complex shapes and designs make them challenging to build and operate, but they have the potential to achieve higher plasma temperatures and densities than tokamak reactors.
Stellarators were first developed in the 1950s, but their complex designs made them difficult to build and operate. However, advances in computer modeling and manufacturing techniques have made them more feasible in recent years.
The Wendelstein 7-X stellarator in Germany is one of the most advanced stellarator reactors in the world. It uses superconducting magnets to create a stable plasma and has achieved temperatures of over 100 million degrees Celsius.
Inertial Confinement Fusion Reactors
Inertial confinement fusion reactors use lasers to compress and heat pellets of hydrogen fuel, creating a miniature fusion explosion.
These reactors are attractive because they can be relatively small and portable, but they require precise targeting and timing to achieve fusion.
Inertial confinement fusion has been used in nuclear weapons for decades, but its use in power generation is still in the experimental phase. The National Ignition Facility in California is one of the most advanced inertial confinement fusion facilities in the world and has made significant progress in recent years.
One of the challenges in using inertial confinement fusion for power generation is finding a way to create a self-sustaining reaction. Currently, the energy required to create the fusion reaction is greater than the energy produced by the reaction.
Magnetized Target Fusion Reactors
Magnetized target fusion reactors use a combination of magnetic fields and plasma compression to achieve fusion reactions.
While they are still in the experimental stage, they hold promise for their potential to be compact and cost-effective fusion reactors.
One of the advantages of magnetized target fusion is that it can use a variety of fuels, including hydrogen, boron, and helium-3. This could make it a more versatile and sustainable form of power generation.
The University of Washington is one of the leading institutions in magnetized target fusion research. Their Fusion Science and Technology Center is dedicated to developing new fusion technologies and advancing our understanding of fusion science.
Conclusion
Fusion reactors hold the promise of abundant and sustainable energy for the future, but they remain challenging to build and operate. As researchers continue to develop new technologies and designs, we may one day see fusion reactors providing clean and limitless energy to the world.