In recent years, neuroscience has been at the forefront of technological development, with remarkable advances being made in areas such as optogenetics, brain-computer interfaces, gene editing, and deep brain stimulation. These technologies have transformed our understanding of the brain and have opened up exciting new possibilities for research, diagnosis, and treatment of neurological disorders.
1. Optogenetics: Shedding Light on Neural Circuits
Optogenetics is a cutting-edge technique that enables scientists to control and modulate the activity of specific neurons in the brain using light. By introducing light-sensitive proteins into these neurons, researchers can selectively activate or inhibit them with unprecedented precision, allowing them to identify and study complex neural circuits that are involved in a wide range of behaviors and brain functions.
The Basics of Optogenetics
The key to optogenetics is the use of microbial opsins, such as Channelrhodopsin-2 (ChR2) and Halorhodopsin (NpHR), which are light-sensitive proteins that are found in algae and bacteria. These proteins can be genetically engineered and inserted into neurons using viral vectors, making them sensitive to specific wavelengths of light.
When light is shone onto these neurons, the opsins activate or inhibit them, triggering a response that can be recorded and analyzed. This allows researchers to probe the neural circuitry of the brain and explore how different neurons and circuits are involved in behaviors such as memory, learning, and decision-making.
One of the most exciting aspects of optogenetics is its ability to target specific populations of neurons within the brain. For example, researchers can use optogenetics to selectively activate or inhibit neurons in the prefrontal cortex, a brain region that is involved in decision-making and social behavior. By doing so, they can study how these neurons contribute to these complex behaviors and potentially develop new treatments for disorders that affect them.
Applications in Neuroscience Research
Optogenetics has already led to many groundbreaking discoveries in neuroscience. For example, it has been used to show that specific populations of neurons in the hypothalamus are involved in regulating hunger and satiety, and that these neurons can be activated or inhibited using light to control feeding behavior in mice.
Moreover, optogenetics has also been applied to investigate the neural basis of psychiatric disorders such as anxiety and depression. By selectively activating or inhibiting neurons in specific brain regions, researchers can identify the neural circuits that are disrupted in these disorders and develop new strategies for treatment.
Another exciting application of optogenetics is in the field of neural prosthetics, where researchers are developing devices that can restore function to damaged or diseased parts of the brain. By using optogenetics to control the activity of neurons in these devices, researchers hope to create more precise and effective treatments for conditions such as Parkinson's disease and epilepsy.

Future Directions and Challenges
Despite its incredible potential, optogenetics still faces several challenges and limitations. One major hurdle is the delivery of the light-sensitive proteins to specific neurons within the brain using viral vectors, which can be difficult and invasive.
Moreover, the effects of optogenetic stimulation can be difficult to interpret, as they may differ from the effects of natural neuronal activity. Additionally, there are concerns about the long-term safety of these technologies, particularly in human applications.
Despite these challenges, the field of optogenetics continues to advance at a rapid pace. Researchers are working to develop new and more precise methods for targeting specific neurons within the brain, as well as exploring new applications of the technology in fields such as neuroengineering and neuroprosthetics. With continued research and development, optogenetics has the potential to revolutionize our understanding of the brain and lead to new treatments for a wide range of neurological and psychiatric disorders.
2. Brain-Computer Interfaces: Bridging the Gap Between Mind and Machine
Brain-computer interfaces (BCIs) are a new class of devices that allow individuals to control electronic devices or prosthetic limbs using their thoughts. These devices work by recording the electrical signals produced by the brain and decoding them using advanced algorithms to translate them into commands for external devices.
Understanding Brain-Computer Interfaces
BCIs use a variety of techniques to record brain activity, including electroencephalography (EEG), which measures the electrical activity of the brain using electrodes attached to the scalp, and intracortical electrodes, which are implanted directly into the brain.
These recordings are then processed using machine learning algorithms that can decode the patterns of neural activity and translate them into commands for external devices such as computers, prosthetic limbs, or even robotic exoskeletons.

Current Applications and Success Stories
BCIs have already shown great promise in a range of applications, including neuroprosthetics, communication, and entertainment. For example, researchers have used BCIs to restore motor function to individuals with spinal cord injuries, allowing them to control prosthetic limbs using their thoughts alone.
Moreover, BCIs have also been used to help individuals with locked-in syndrome or severe communication disorders to communicate with their loved ones and caregivers, by enabling them to select letters or words on a computer screen using their thoughts.
Ethical Considerations and Future Potential
Despite their potential benefits, BCIs also raise several ethical concerns, particularly with regards to privacy, autonomy, and human enhancement. For example, there are concerns about the potential misuse of these technologies for surveillance or mind-reading purposes, as well as the potential for them to be used as a form of cognitive enhancement or coercion.
However, with careful consideration and regulation, BCIs have the potential to revolutionize many aspects of human life, from healthcare to entertainment.
3. CRISPR-Cas9: Revolutionizing Gene Editing in Neuroscience
CRISPR-Cas9 is a powerful gene-editing technology that allows researchers to make precise changes to DNA sequences in living cells. This technology has transformed the field of molecular biology and has opened up new avenues for research into the genetic basis of neurological disorders.
The Science Behind CRISPR-Cas9
CRISPR (Clustered Regularly Interspaced Short Palindromic Repeats) refers to a naturally occurring bacterial immune system that recognizes and destroys foreign DNA sequences. Cas9 (CRISPR-Associated Protein 9) is a protein that acts as a molecular scissors, cutting the DNA at specific target sites that are complementary to a small RNA molecule known as a guide RNA (gRNA).
By introducing a customized gRNA along with the Cas9 protein, researchers can direct the enzyme to cut a specific location in the genome, allowing them to insert or delete specific genes or to repair aberrant genetic sequences that cause disease.
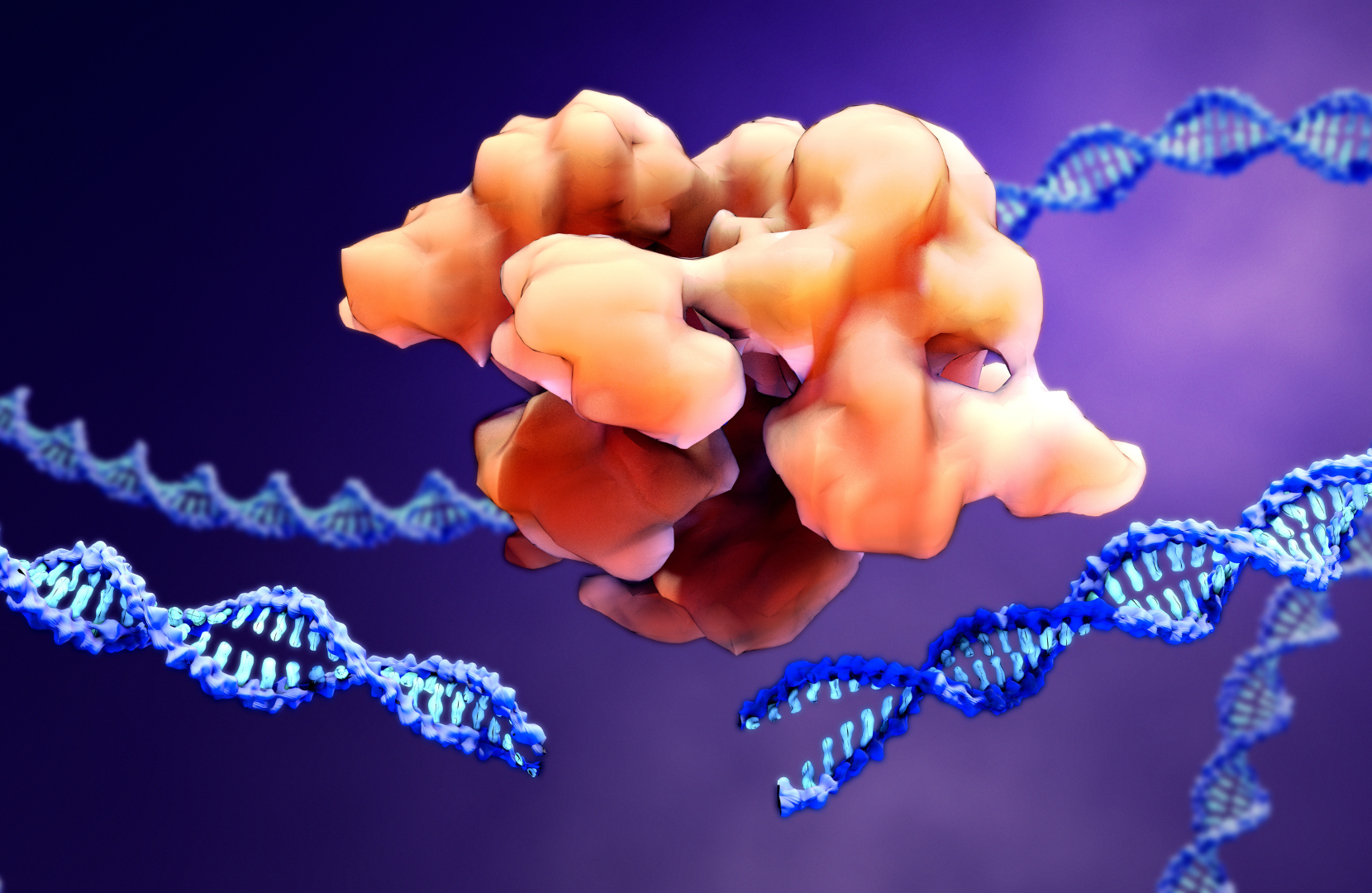
Applications in Neurological Disorders
CRISPR-Cas9 has already been used to investigate the genetic basis of many neurological disorders, such as Huntington's disease, Alzheimer's disease, and autism spectrum disorders. By using this technology to create animal models of these diseases that replicate the human genetic mutations, researchers can explore the underlying molecular mechanisms and identify potential therapeutic targets.
Furthermore, CRISPR-Cas9 has also been used to develop new strategies for gene therapy, such as replacing defective genes or correcting genetic mutations in living cells.
Ethical Debates and Future Prospects
CRISPR-Cas9 also raises several ethical concerns, particularly with regards to gene editing in human embryos or germline cells, which could have long-lasting implications for future generations. There are also concerns about the potential for off-target effects or unintended consequences of gene editing, as well as the potential for eugenics or attempts to create "designer babies."
However, with careful regulation and ethical oversight, CRISPR-Cas9 has the potential to revolutionize the field of molecular biology and to provide new avenues for treatment and cure of neurological disorders.
4. Deep Brain Stimulation: A New Era of Treatment for Neurological Disorders
Deep brain stimulation (DBS) is a surgical procedure that involves the implantation of electrodes into specific regions of the brain to modulate neural activity. This technique has shown great promise in the treatment of neurological disorders such as Parkinson's disease, depression, and epilepsy.
Principles of Deep Brain Stimulation
The key to DBS is the precise targeting of specific brain regions that are involved in the disorder. Once the electrodes are implanted, they are connected to a pulse generator that delivers electrical impulses to the brain at a specific frequency and intensity.
These electrical impulses can modulate the activity of neurons in the brain, helping to restore or regulate abnormal patterns of neural activity that underlie the neurological disorder.
Clinical Applications and Success Stories
DBS has already been used successfully to treat a range of neurological disorders, including Parkinson's disease, essential tremor, dystonia, and obsessive-compulsive disorder. In many cases, DBS has been shown to be more effective than conventional drug therapies, with fewer side effects and a better quality of life for patients.
Moreover, DBS has also been used to map the neural circuitry of the brain, allowing researchers to identify the neural pathways that are involved in these disorders and to develop new strategies for treatment.
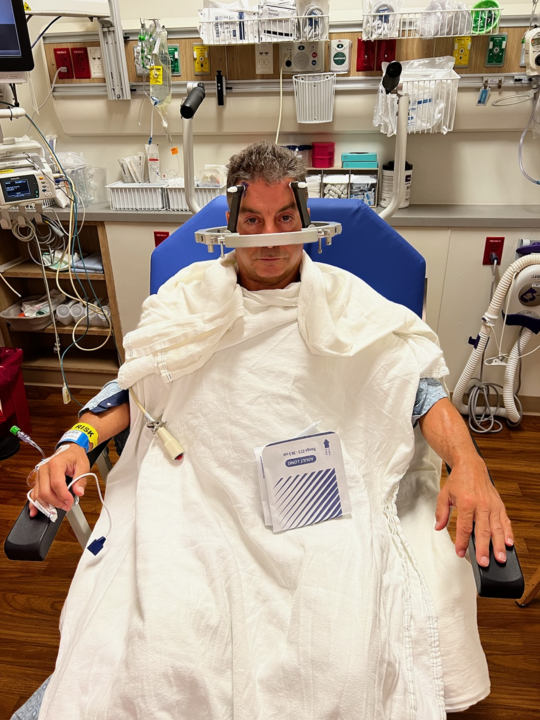
Risks, Limitations, and Future Developments
Despite its potential benefits, DBS is not without risks. The surgical procedure itself can carry some risks, such as infection or hemorrhage, and there may also be long-term effects on cognitive function or behavior.
Furthermore, the precise mechanisms of DBS are not fully understood, and there is still much to learn about how it modulates neural activity and how different brain regions interact with each other.
However, with ongoing research and development, DBS has the potential to become a mainstream treatment option for many neurological disorders, offering hope to millions of individuals around the world.
Conclusion
These are just some of the latest technologies that are transforming the field of neuroscience and opening up new possibilities for research, diagnosis, and treatment of neurological disorders. Each of these technologies has its own unique strengths and limitations, and there is still much to learn about how they interact with the complex neural circuitry of the brain.
However, with ongoing research and innovation, these technologies are sure to continue to shape the future of neuroscience, paving the way for new discoveries and new therapies that could change the lives of millions of people around the world.