All organisms, from the most complex to the single-celled, follow a life cycle. In humans, this cycle begins while the child is still in its mother's womb and ends, when not interrupted, with “death from old age” (that means death caused by one or more diseases connected to old age). Based on the medical technology and knowledge of our time, people in Europe live on average up to 81.3 years old. In the future, we may live several years, decades or even centuries longer. In order to achieve this goal, we not only have to cure the diseases that induce death. We must first understand how aging works and then find ways to slow it down or even stop it. So let’s have a look at what happens to our bodies when we age.
‍

‍
9 Hallmarks of Aging
‍
Aging is a very complex progressive process that leads an organism (in our case a person) to impared functions and increases its vulnerability to death. Age is in fact at the top of the list of risk factors for conditions such as heart diseases and cancers - currently the leading causes of death worldwide.
Over the last few decades, aging research has experienced an unprecedented advance, particularly with the discovery that aging is controlled, at least to some extent, by genetic pathways and biochemical processes. Different research has outlined 9 hallmarks that represent common denominators of aging in different organisms. Their relationship is interconnected and the aim of future medical technology will be to understand these connections and tackle all different aspects of aging.
‍

‍
Genomic instability
‍
Every single cell that makes up our bodies (and we have billions and billions of them) contains a complete instruction set that, alone, could rebuild us from scratch. This instruction set is called the genome and consists of nucleotide sequences of DNA. Among other things, our genome contains the instructions for the creation of proteins, which are essential for the proper functioning of our cells.
Normally, our somatic cells split into two genetically identical cells: the genetic material is replicated and possible mistakes or damages are repaired. As the process is not perfect, mutations can occur during cell division. Fortunately, our bodies have intrinsic DNA repair mechanisms that can fix most of these errors and other DNA damages due to external genotoxic factors (such as, to name one, ultraviolet radiation from the sun).
Now, these mutations occur throughout our lives. But the problem is that, as we age, our repair mechanisms weaken and are no longer able to repair mutations efficiently. Genomic instability is the term we use when there is an increased tendency for genome alterations during cell division. The mutated genome may produce broken proteins. The result is dysfunctional cells that accumulate over the years.
While a single defective cell is not problematic, when there are many within the same tissue, they can compromise the functionality of the entire tissue. That said, if we could find a way to prevent our DNA repair mechanisms from weakening with age or if we could support their crucial work with specific drugs, we may find a way to tackle genome instability.
‍
Telomere attrition
‍
Have you ever heard about telomeres? Telomeres are disposable parts of DNA that don’t contain any useful genetic information. They sit at the end of chromosomes (DNA molecules that can be found in the nucleus of each of our cells).
Telomeres' role in cell replication is crucial. In fact, every time a cell replicates, a small part of the edges of the DNA strings, the telomeres, gets lost in the process. If telomeres didn’t exist, the main part of the chromosome would shorten instead and genes essential for life would get lost. Telomere attrition is the name used for the shortening of the telomeres after each cell division.
Since pieces of telomeres are lost after each replication, the new chromosomes will have shorter and shorter telomeres. Eventually, the DNA will be left unprotected and cells won’t be able to duplicate anymore. Once a cell can’t replicate anymore, it becomes senescent or it kills itself. Because of telomere attrition, over the years our body becomes unable to create new cells and to fight diseases.
Yet, there could be solutions for telomere attrition.
- First of all, our stem cells (cells that don’t have a specialized function) produce an enzyme called telomerase that rebuilds the pieces of telomeres lost in the cell division process. Specialized cells are not able to produce this useful enzyme themselves but we could activate them through telomerase therapy. A company working on this is Telocyte: using telomerase, they are rebuilding the telomeres of brain cells that became dysfunctional because of Alzheimer’s.
- Secondly, cancer cells can rebuild their telomeres indefinitely. We don’t know exactly how they do it but, if we could understand the mechanism, we may be able to replicate it for our own cells.
‍
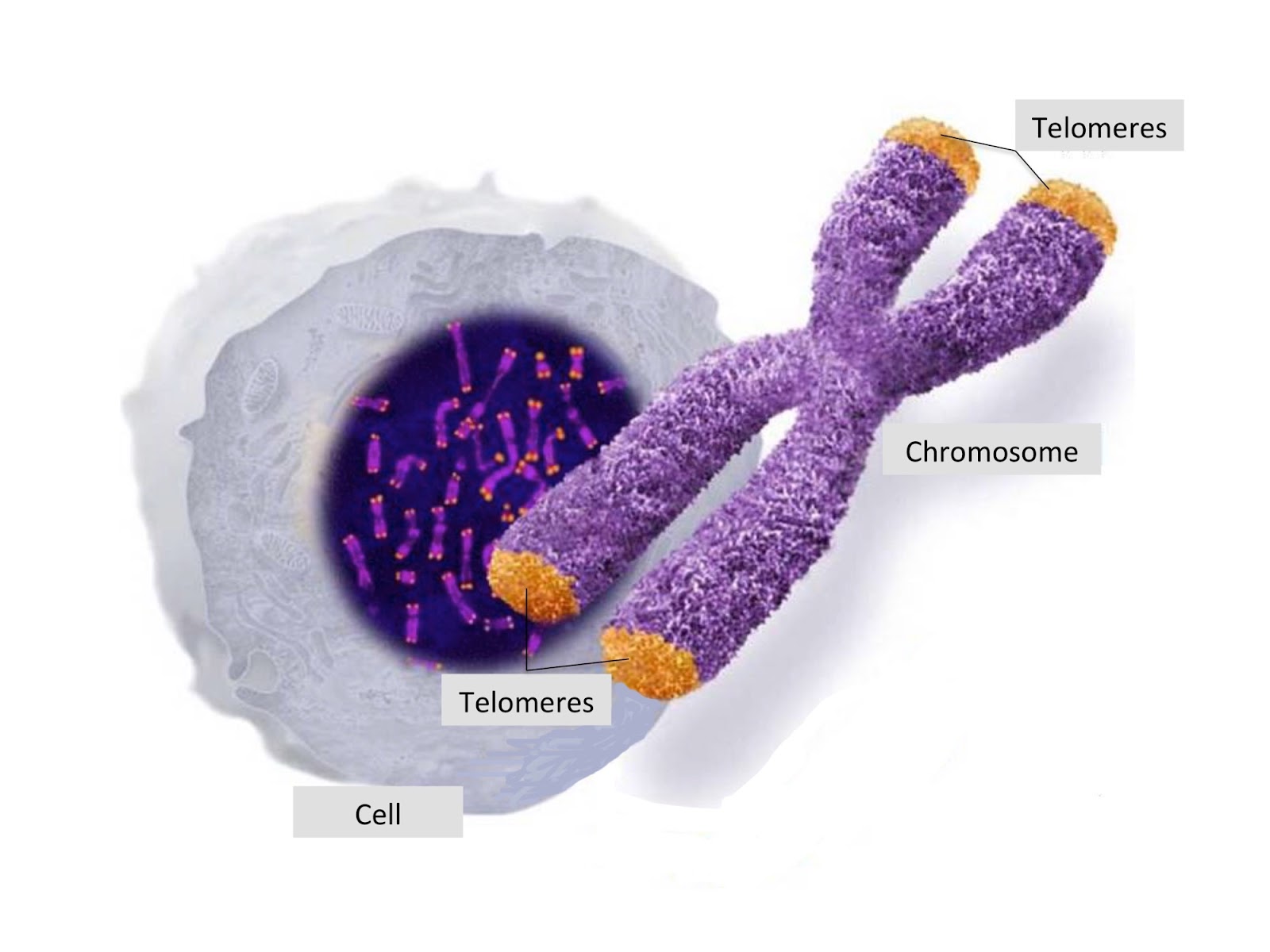
‍
Epigenetic alterations
‍
As seen above, all the cells in our body, regardless of their role, contain the same genome. However, these cells have different roles and perform different functions (e.g. brain cells and muscle cells). How is this possible?
The answer can be found in the so-called epigenetic changes. These are reversible changes (caused by chemical compounds) that activate and deactivate some parts of our DNA, without changing the genetic code sequence. While all cells have the same genome, specialized cells have different epigenomes that influence the production of specific proteins, defining the cells’ function. Epigenetic changes happen normally in the human body. For example, we are born with a high number of stem cells, which specialize in different tissues by activating or not activating the genes contained in the DNA strings.
During the years, several external factors, such as nutrients, pollution, toxicants (smoke), stress and inflammation can trigger epigenomic alterations. Genes that should be inactive are activated and vice versa, creating malfunctioning cells.
Luckily, there may be a way to eliminate epigenetic alterations and return the genome to its original state. In 2016 scientists at the Salk Institute managed to reset the epigenome of mice by exposing them to a cocktail of chemicals called Yamanaka factors. This procedure can turn specialized cells into unspecialized stem cells, resetting their epigenome. Could we use a similar procedure to rejuvenate human cells?
‍
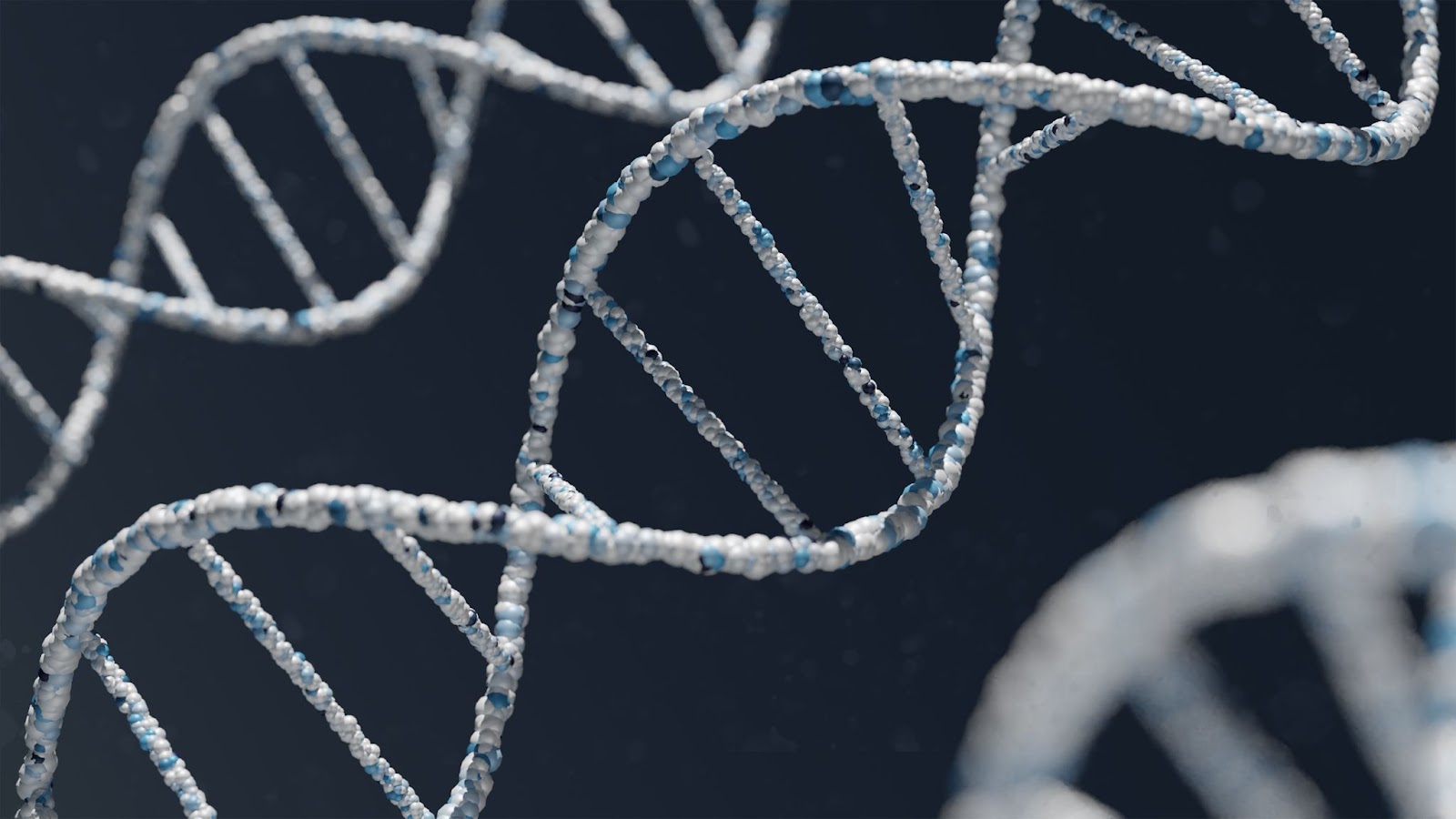
‍
Loss of proteostasis
‍
Proteins allow our cells to do everything, from sensing the environment, digesting the food, contracting a muscle, sending electrical signals etc.
What we define as loss of proteostasis is the accumulation, over the years, of damaged proteins, both inside and outside our cells. Our body repair mechanisms can’t do much to fix these damaged proteins and their accumulation can lead us to several diseases: Alzheimer’s, Parkinson’s, metabolic disorders and heart failure.
How do we fix this problem? There are 3 approaches:
- Slow down the accumulation of damaged proteins.
- Counteract their negative effects.
- Destroy or remove these proteins.
There are several studies for each of these approaches. A successful example of the last approach was applied to treat Alzehimer’s in Grifols' recent Alzheimer Management by Albumin Replacement (“AMBAR”) study. In this study, the researchers drew blood from the patients’ body and cleansed it of waste and one of the damaged proteins (amyloid beta) that causes the disease. Once reinjected, the cleaned blood drained this harmful protein from the brain, slowing the progress of the disease by 50%. While this technique is not a final solution, it shows that medical procedures and drugs can help in tackling the loss of proteostasis.
‍
Deregulated nutrient sensing
‍
To function, all cells in our body require nutrients that are absorbed from the food we eat. Our cells have specific mechanisms that allow them to sense when nutrients are abundant (and the cells accelerate growth and metabolism) and when they are scarce (and it’s therefore time to slow down).
These mechanisms also help to keep the level of nutrients in our bloodstream within a healthy range. For example, cells in the pancreas respond to the rise in blood sugar levels by releasing the hormone insulin, which signals to cells to absorb the sugar from our bloodstream. If they didn’t, the high amount of sugar would damage several organs. When we age, these sensing mechanisms start to malfunction (becoming underlying causes of age-related to obesity, type 2 diabete and metabolic diseases).
If we could understand our nutrient sensing mechanism better, we could possibly extend our lifespan and healthspan. Our cells’ activity could in fact be regulated with specific drugs, so that the metabolic rate decreases and with it the amount of damage accumulated over the years.
‍
Mitochondrial dysfunction
‍
Once the nutrients get inside our cells, small organelles called mitochondria convert them into energy that the cells can use to function and survive. This energy is called adenosine triphosphate or ATP and, for this reason, mitochondria are considered the “powerhouses” of the cells.  Mitochondria have their own DNA, different from the DNA contained in the nucleus, which helps them create the proteins they need for their specific function.
Mitochondrial dysfunction can be caused by several factors. If the membrane structure of the mitochondria is altered, if the proteins located on the same membrane are broken or if the mitochondrial DNA is damaged, the mitochondria won’t be able to normally produce the energy (ATP) necessary for the cells to function. When mitochondrial function declines, cells and whole organs can deteriorate too (leading to diseases such as diabetes, deafness, blindness and neural dysfunctions like Alzheimer’s and Parkinson’s).
Now, our body has intrinsic repair mechanisms. Unfortunately, these mechanisms are often not very effective in repairing damaged mitochondria. As for all hallmarks of aging, various studies are being carried out to find possible solutions that will enable us to extend our lifespan. One drug for mitochondrial dysfunction, currently in the early stages of human testing, is J147. The aim of this drug is to optimize the energy production of the mitochondria so that, even when reduced, it is still sufficient for cellular functioning.
‍
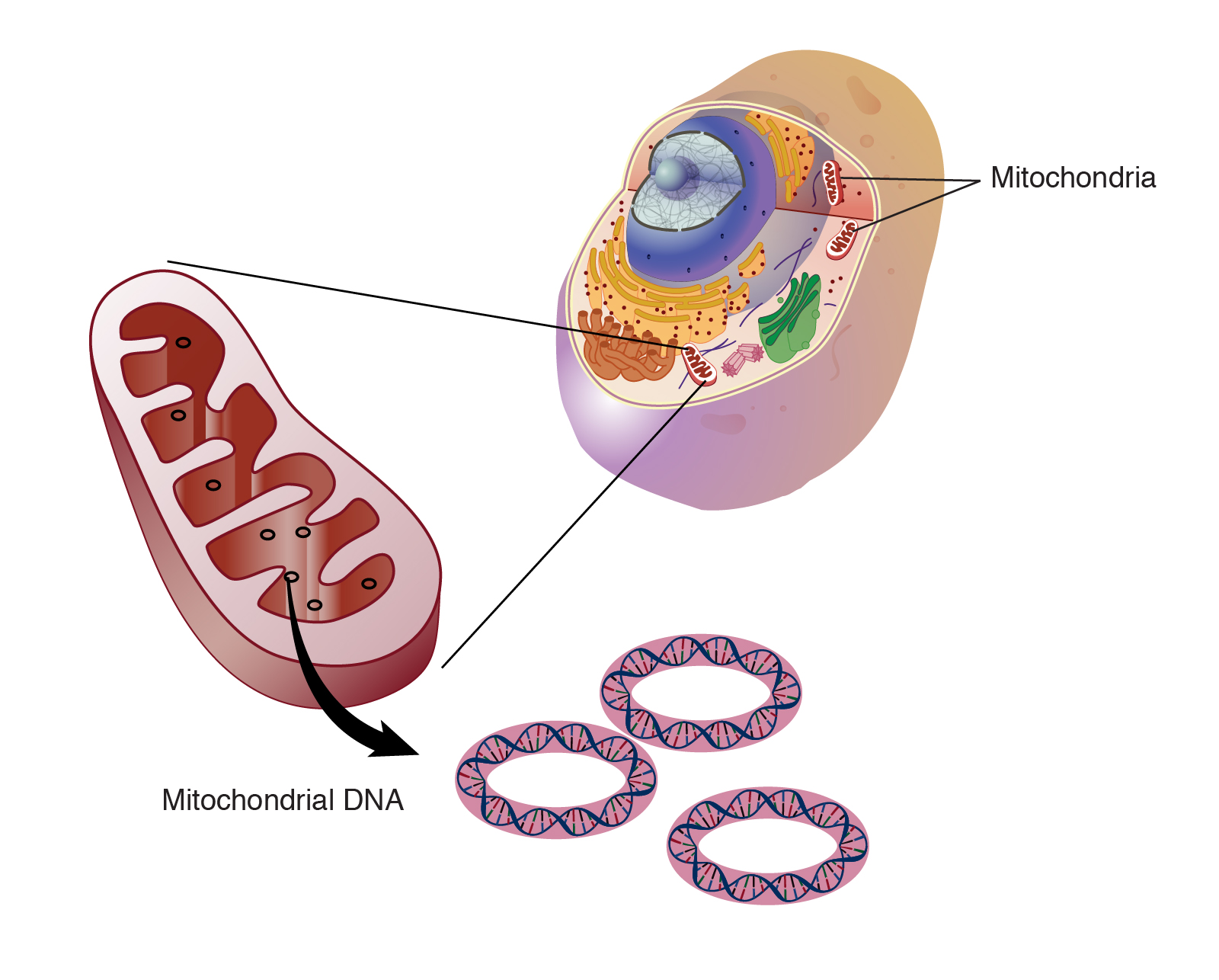
‍
Cellular senescence
‍
As we age, our cells age with us. In fact, after about 50 replications, our cells start breaking down and lose their function. In addition to that, several stress-inducing factors can cause cellular senescence: DNA damage, epigenetic alterations, smoke and ultraviolet rays from the sun.
When the telomeres are exhausted, the cells are unable to divide and wait for repair mechanisms to fix them. When these mechanisms are unable to do so (which happens more often with age), the cells die or become senescent.
When we are young, senescent cells help us deal with tissue repair, wound regeneration and protect us from cancer. But, as they accumulate over the years, they begin to damage neighboring cells. In fact, senescent cells produce chemicals that can cause inflammation, interfere with cellular growth and activate and deactivate genes in other cells’ DNA.
Is there a way we can destroy senescent cells without damaging healthy cells? In the last few years, scientists have developed several cell-destroying drugs, called senolytics. While most of these drugs are still in their early stages, we could be optimistic about the fact that some remedies for cell senescence are about to be found.
‍
Stem cell exhaustion
‍
Our bodies need new cells continuously. To meet this need, our cells split several times into identical copies. As we have said, these divisions are limited in specialized cells because of telomere attrition. In stem cells, the possibility of replication is almost infinite, thanks to the enzyme telomerase, which replenish the telomeres.
Unfortunately, several hallmarks of aging affect the availability and activity of stem cells, producing the phenomenon we call stem cell exhaustion. For example, the accumulation of DNA damage can make blood stem cells work less efficiently. The decline of stem cell populations can lead to several diseases and conditions such as frailty, muscle loss, osteoporosis, anemia and poor immune function.
Currently, there are several stem cell therapies that, through the injection of stem cells, can prevent disease from happening and even help the creation of new tissue in the diseased organs.
‍
Altered intercellular communication
‍
Now we come to the last hallmark of aging. Organs, tissues and systems that make up the human body are interrelated and their functioning influences and depends on these connections. To communicate and work with each other, cells use a network of chemical signals about which we still know very little.
Altered intercellular communication accounts for changes in the communication system. The first sign of this altered communication can be seen in chronic inflammation caused by aging (or inflammaging), which can contribute to the development of obesity and type 2 diabetes. Moreover, chronic inflammation can negatively influence the activity of stem cells and inhibit the immune system.
To add to the problem, senescent cells also produce chemicals that increase the inflammation. Since the problem is strictly related, finding a solution for cellular senescence would also improve the situation for chronic inflammation.
‍
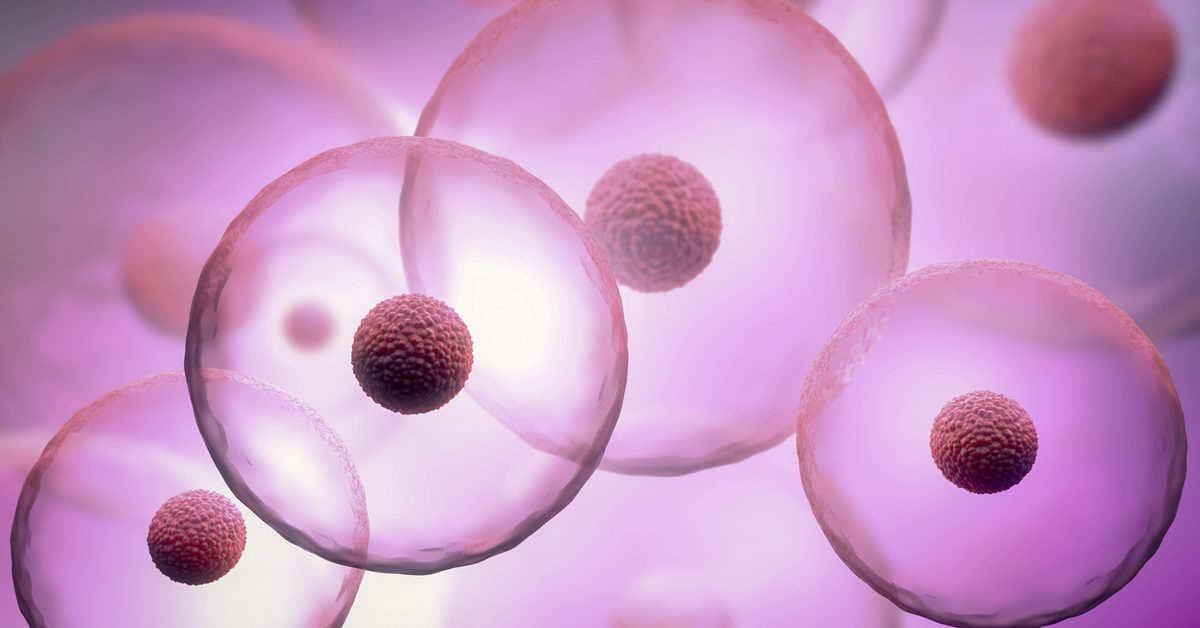
‍
Conclusion
‍
The aging process consists of many interconnected processes. While it might seem that this complexity is a negative feature in understanding how aging works, it can also be used to our advantage. Indeed, finding solutions for one of these hallmarks would positively affect the other hallmarks as well.
Medical technology is making tremendous progress in discovering solutions for aging. These solutions may allow us to live much longer in the future.
If you want a chance to see what the future holds for us, sign up here and join us in this journey! If you have any questions about Biostasis, feel free to schedule a call with us.