CRISPR, which stands for Clustered Regularly Interspaced Short Palindromic Repeats, is a revolutionary gene editing technology that has garnered significant attention in recent years. This powerful tool allows scientists to precisely modify and edit DNA, opening up new possibilities for advancements in medicine, agriculture, and various other fields.
Understanding the Basics of CRISPR
In order to fully grasp the potential of CRISPR, it is essential to dive into the fundamental concepts behind this groundbreaking technology.
CRISPR (Clustered Regularly Interspaced Short Palindromic Repeats) is derived from the defense mechanism found in bacteria to protect against viral infections. It consists of two essential components: the Cas9 protein and guide RNAs. The Cas9 protein acts as molecular scissors, capable of cutting DNA at specific target sites, while the guide RNAs provide the necessary instructions to direct Cas9 to the desired genetic sequence.
The science behind CRISPR is truly fascinating. Bacteria use this system to store genetic information from previous viral infections and use it to recognize and destroy viral DNA. The CRISPR system consists of short DNA sequences called repeats, which are separated by unique DNA sequences called spacers. These spacers are derived from viral DNA and serve as a memory bank for the bacteria. When the bacteria encounter a virus again, the CRISPR system uses the spacers to guide the Cas9 protein to the viral DNA and cut it, effectively neutralizing the virus.
The Science Behind CRISPR
CRISPR technology has revolutionized the field of genetic engineering. With its ability to precisely edit DNA, scientists can now target and modify specific genes, opening up a world of possibilities for treating genetic diseases, developing new therapies, and improving agricultural practices.
One of the key features of CRISPR is its versatility. Unlike previous gene-editing techniques, CRISPR is relatively simple and cost-effective, making it accessible to researchers around the world. It has been successfully used in a wide range of organisms, from bacteria to plants and animals, including humans. This versatility has sparked immense excitement and optimism within the scientific community.
History and Development of CRISPR
The history and development of CRISPR technology is a testament to the power of scientific curiosity and collaboration. The initial discovery of CRISPR-like sequences in bacteria took place in the 1980s when scientists noticed these unique repetitive DNA sequences. However, it wasn't until 2012 that researchers Jennifer Doudna and Emmanuelle Charpentier demonstrated the potential of CRISPR for precise gene editing.
Since then, CRISPR has rapidly advanced, with further developments making it more efficient and versatile. Scientists have made significant improvements to the CRISPR system, enhancing its precision, reducing off-target effects, and expanding its applications. They have also explored different variations of CRISPR, such as CRISPR-Cas12 and CRISPR-Cas13, each with its own unique capabilities.
The impact of CRISPR technology extends far beyond the laboratory. It has sparked ethical debates and raised important questions about the implications of gene editing. As scientists continue to push the boundaries of what is possible with CRISPR, it is crucial to engage in thoughtful discussions and consider the ethical and societal implications of this powerful tool.
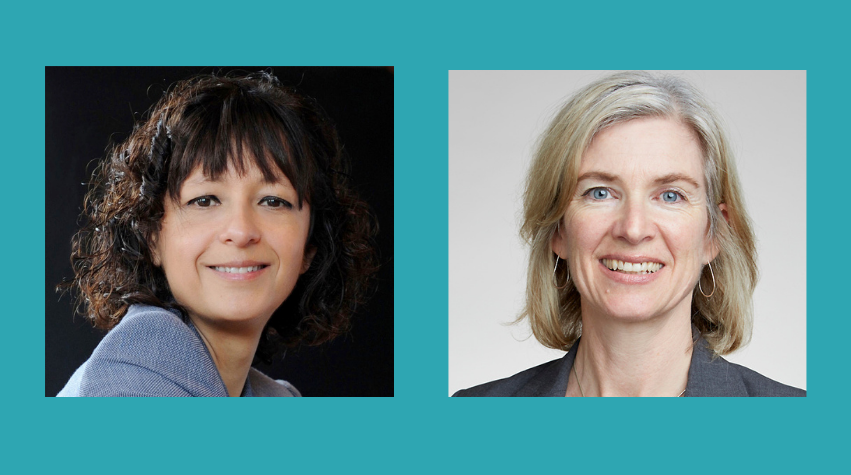
The Components of CRISPR
Now that we have a foundational understanding of CRISPR, let's take a closer look at its individual components and their respective roles in the gene editing process.
CRISPR technology has revolutionized the field of genetic engineering, allowing scientists to make precise modifications to an organism's DNA. At the heart of this powerful tool are two key components: Cas9 and guide RNAs.
The Role of Cas9 in CRISPR
The Cas9 protein is a key player in CRISPR technology. It is responsible for identifying and cutting the DNA at the designated target site, enabling precise modifications to occur. Cas9 can be thought of as the molecular scissors that make gene editing possible.
But how does Cas9 know where to make these cuts? This is where guide RNAs come into play.
Guide RNAs: The Navigation System of CRISPR
In order for Cas9 to find its target site, it requires a set of guide RNAs. These guide RNAs provide the necessary instructions for Cas9, directing it to the specific location on the DNA where the desired changes need to be made. It's as if guide RNAs act as the navigation system for CRISPR, ensuring that the gene editing process takes place at the right location.
Guide RNAs are short RNA molecules that are complementary to the target DNA sequence. They are designed in the lab and can be customized to match the specific DNA sequence that scientists want to edit. These guide RNAs bind to the DNA and guide Cas9 to the correct location, like a GPS guiding a driver to a destination.
Once Cas9 reaches its target site, it uses its molecular scissors to cut the DNA. This cut triggers the cell's natural repair mechanisms, which can be harnessed to introduce specific changes to the DNA sequence. Scientists can then insert, delete, or modify genes as desired, opening up a world of possibilities for genetic engineering.
It's important to note that CRISPR technology is not limited to just Cas9 and guide RNAs. There are other variations of the CRISPR system, each with its own unique components and mechanisms. For example, some CRISPR systems use different enzymes instead of Cas9, while others use different types of guide RNAs. These variations allow scientists to tailor the CRISPR technology to suit their specific research needs.
.png)
The Process of CRISPR
With an understanding of the individual components, let's explore the step-by-step process through which CRISPR edits genes.
The process of CRISPR gene editing involves several intricate steps that work together to modify specific genetic sequences. It begins with the design and creation of guide RNAs, which are synthetic molecules that are specifically tailored to target a particular gene sequence. These guide RNAs are crucial in directing the CRISPR system to the desired location in the DNA.
Once the guide RNAs are synthesized, they are combined with the Cas9 protein, forming a complex. Cas9 is an enzyme that acts as a pair of molecular scissors, capable of precisely cutting the DNA strands. The guide RNAs serve as the GPS, guiding Cas9 to the target site within the genome.
When the CRISPR complex reaches the target site, Cas9 cuts the DNA, creating a double-stranded break. This break triggers the cell's natural repair mechanisms, which kick into action to fix the damaged DNA. There are two primary repair pathways that can be activated: non-homologous end joining (NHEJ) and homology-directed repair (HDR).
In the case of NHEJ, the broken DNA ends are directly rejoined, often resulting in small insertions or deletions of genetic material. This repair mechanism can be harnessed to disrupt specific genes or introduce small changes in the DNA sequence.
On the other hand, HDR is a more precise repair pathway that can be utilized to insert specific DNA sequences at the site of the double-stranded break. Scientists can provide a DNA template with the desired sequence, and the cell's repair machinery uses this template as a blueprint to accurately repair the DNA, incorporating the new genetic material.
After the repair process is complete, the modified DNA is integrated into the cell's genome, resulting in the desired gene modifications. This could involve correcting disease-causing mutations, introducing beneficial traits, or studying the function of specific genes.
.png)
The Precision of CRISPR Technology
While CRISPR has ushered in a new era of gene editing, it is important to note that the technology is not without limitations. Although CRISPR is highly precise in targeting specific sequences, off-target effects can still occur. These off-target effects refer to unintended changes in DNA sequences that are similar to the target site but not the intended target itself.
Scientists are continually working to refine and improve the precision of CRISPR to minimize these unintended changes. Various strategies, such as modifying the Cas9 enzyme or optimizing the design of guide RNAs, are being explored to enhance the specificity of CRISPR gene editing.
Additionally, researchers are developing advanced techniques to detect and analyze potential off-target effects. These methods include high-throughput sequencing and computational algorithms that can identify any unintended alterations in the genome, allowing scientists to assess the accuracy of CRISPR-mediated gene edits.
By addressing these challenges and optimizing the CRISPR technology, scientists aim to unlock the full potential of gene editing for various applications, ranging from healthcare and agriculture to basic research and biotechnology.
Applications of CRISPR
Now that we have a solid understanding of how CRISPR works, let's explore the wide range of applications this technology holds.
CRISPR in Medicine
One of the most promising areas of CRISPR application is in the field of medicine. Researchers believe that CRISPR could revolutionize the treatment of genetic disorders, making it possible to correct disease-causing mutations at their source. Additionally, CRISPR holds potential for developing targeted cancer therapies, creating personalized medicines, and combating infectious diseases.
.png)
CRISPR in Agriculture
The agricultural industry is also poised to benefit from CRISPR technology. By employing gene editing techniques, scientists can create crops that are more resistant to pests, diseases, and environmental stresses. This could potentially increase crop yields, reduce the use of pesticides, and contribute to more sustainable agriculture practices.
Other Potential Uses of CRISPR
Beyond medicine and agriculture, CRISPR has the potential to impact various other fields. It could be utilized in bioengineering to produce renewable energy sources, in environmental conservation to protect endangered species, and even in the manufacturing industry to optimize the production of desirable substances.
Ethical Considerations of Using CRISPR
While the potential of CRISPR is undeniably exciting, its use also brings about a range of ethical considerations that must be carefully examined.
The Debate Over Gene Editing
One of the primary ethical concerns surrounding CRISPR is the debate over the boundaries of gene editing. Some argue that the technology could be used to create "designer babies" or to make modifications that could have unintended consequences for future generations. Balancing the potential benefits of gene editing with the ethical implications is a complex and ongoing discussion.
Potential Risks and Challenges of CRISPR
Another aspect that demands careful consideration is the potential risks and challenges associated with CRISPR. It is crucial to minimize off-target effects and ensure the safety of editing procedures. Additionally, the unequal access to this technology across different populations and the potential for unintended consequences must be closely monitored.
Conclusion
CRISPR represents a groundbreaking advancement in gene editing technology. With its precise and versatile capabilities, CRISPR holds immense potential for revolutionizing various industries, including medicine, agriculture, and beyond. However, as we continue to explore the possibilities of this powerful tool, it is crucial to address the ethical considerations and ensure responsible and accountable use of CRISPR for the betterment of society.